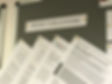
Our Publications
Selected Publications
Multiple myeloma (MM) is an incurable plasma cell malignancy primarily localized within the bone marrow (BM). Myeloma plasma cells, like many other cancer cells, change their metabolism in response to internal and external stimuli. The main metabolic alterations of MM cells include deregulated glycolysis (commonly associated with enhanced uptake and utilization of glucose), lipid metabolism dysregulation, as well as deregulated mitochondrial respiration (commonly associated with the deregulated formation of reactive oxygen species). Over the past decade, the discovery of novel methodologies and the commercialization of sophisticated instrumentation and reagents have facilitated the detection of real-time changes in cellular bioenergetics. Of those, the Seahorse™ extracellular flux (XF) analyzer has been widely used to evaluate the glycolytic flux and mitochondrial respiration in many cell types. While adherent cell lines are easy to use with this technology, non-adherent suspension cells are more difficult to handle especially when their metabolic activities are being investigated in response to drug treatment. Here, we provide an integrated protocol that allows the detection of extracellular acidification rate (ECAR) of live myeloma plasma cells in response to chemotherapeutic drugs. Our optimized protocol consists of treating myeloma cells with cytotoxic drug of interest in a standard culture plate prior to the real-time analysis in the XF analyzer. Furthermore, we provide results of experiments in which the metabolic activities of myeloma cells in response to cytotoxic treatment were compared between the manufacturer’s basic procedure and our optimized protocol. Our observations suggest that our integrated protocol can be used to achieve consistent, well-standardized results and thus it may have broad applications in studies focusing on the characterization of metabolic events in non-adherent suspension cells.
Aerobic glycolysis has been commonly linked to cell proliferation, especially in cancer cells where it serves to generate sufficient energy and biosynthesis of new cell constituents needed for cell growth and division. The M2 isoform of pyruvate kinase (PKM2) catalyzes the last reaction of the glycolytic process. PKM2 promotes the transfer of a phosphate group from phosphoenolpyruvate (PEP) to ADP, generating ATP and releasing pyruvate. This rate-limiting reaction relies therefore on the enzymatic activity of PKM2. The switching between the high- and low-activity states of PKM2 is subjected to a combination of allosteric mechanisms and fine-tuned regulation by oncogenes and tumor suppressor genes. These regulatory mechanisms involve primarily post-translational modifications of PKM2. Recent findings suggest that phosphorylation contributes to the regulation of PKM2 activity.
Here, we describe an in vitro kinase assay we used to assess PKM2 phosphorylation by c-Jun N-terminal kinase (JNK), a master regulator of apoptosis, cell proliferation, and differentiation. While the use of phospho-specific antibodies gives information in terms of measuring the effects of a given kinase on its substrate, specific antibodies for newly identified phospho-groups are not readily available. The in vitro kinase assay allows the immediate measuring of phosphorylation of any substrate of interest. Although there are several options that do not use radioactive materials, we continue to rely on this biochemical method for robust quantitation of results. More interestingly, this protocol can be easily adapted to measure the activity of other kinases by using their specific substrates.
Intrahepatic cholangiocarcinoma (ICC) is a highly aggressive type of liver cancer in urgent need of treatment options. Aberrant activation of the c-Jun N-terminal kinase (JNK) pathway is a key feature in ICC and an attractive candidate target for its treatment. However, the mechanisms by which constitutive JNK activation promotes ICC growth, and therefore the key downstream effectors of this pathway, remain unknown for their applicability as therapeutic targets. Our aim was to obtain a better mechanistic understanding of the role of JNK signaling in ICC that could open up therapeutic opportunities.
Using loss-of-function and gain-of-function studies in vitro and in vivo, we show that activation of the JNK pathway promotes ICC cell proliferation by affecting the protein stability of peptidyl-prolyl cis-trans isomerase NIMA-interacting 1 (PIN1), a key driver of tumorigenesis. PIN1 is highly expressed in ICC primary tumors, and its expression positively correlates with active JNK. Mechanistically, the JNK kinases directly bind to and phosphorylate PIN1 at Ser115, and this phosphorylation prevents PIN1 mono-ubiquitination at Lys117 and its proteasomal degradation. Moreover, pharmacological inhibition of PIN1 through all-trans retinoic acid, a Food and Drug Administration–approved drug, impairs the growth of both cultured and xenografted ICC cells.
Conclusions
Our findings implicate the JNK-PIN1 regulatory axis as a functionally important determinant for ICC growth, and provide a rationale for therapeutic targeting of JNK activation through PIN1 inhibition.
The incidences of liver fibrosis are growing at a rapid pace and have become one of the leading causes of end-stage liver disease. Although TGF-b1 is known to play a prominent role in transforming cells to produce excessive extracellular matrix that lead to hepatic fibrosis, the therapies targeting TGF-b1 have achieved very limited clinical impact. This study highlights motor protein myosin-1c-mediated mechanisms that serve as novel regulators of TGF-b1 signaling and fibrosis.
INVITED EDITORIAL COMMENT (EXPERTS OPINIONS)
This is an invited editorial comment to express the opinions on a published article by Yan-Zhou Zhang and colleagues in Hepatology.
Most tumor cells reprogram their glucose metabolism as a result of mutations in oncogenes and tumor suppressors, leading to the constitutive activation of signaling pathways involved in cell growth. This metabolic reprogramming, known as aerobic glycolysis or the Warburg effect, allows tumor cells to sustain their fast proliferation and evade apoptosis. Interfering with oncogenic signaling pathways that regulate the Warburg effect in cancer cells has therefore become an attractive anticancer strategy. However, evidence for the occurrence of the Warburg effect in physiological processes has also been documented. As such, close consideration of which signaling pathways are beneficial targets and the effect of their inhibition on physiological processes are essential. The MAPK/ERK and MAPK/JNK pathways, crucial for normal cellular responses to extracellular stimuli, have recently emerged as key regulators of the Warburg effect during tumorigenesis and normal cellular functions. In this review, we summarize our current understanding of the roles of the ERK and JNK pathways in controlling the Warburg effect in cancer and discuss their implication in controlling this metabolic reprogramming in physiological processes and opportunities for targeting their downstream effectors for therapeutic purposes
Most tumour cells use aerobic glycolysis (the Warburg effect) to support anabolic growth and evade apoptosis. Intriguingly, the molecular mechanisms that link the Warburg effect with the suppression of apoptosis are not well understood. In this study, using loss-of-function studies in vitro and in vivo, we show that the anti-apoptotic protein poly(ADP-ribose) polymerase (PARP)14 promotes aerobic glycolysis in human hepatocellular carcinoma (HCC) by maintaining low activity of the pyruvate kinase M2 isoform (PKM2), a key regulator of the Warburg effect. Notably, PARP14 is highly expressed in HCC primary tumours and associated with poor patient prognosis. Mechanistically, PARP14 inhibits the pro-apoptotic kinase JNK1, which results in the activation of PKM2 through phosphorylation of Thr365. Moreover, targeting PARP14 enhances the sensitization of HCC cells to anti-HCC agents. Our findings indicate that the PARP14-JNK1-PKM2 regulatory axis is an important determinant for the Warburg effect in tumour cells and provide a mechanistic link between apoptosis and metabolism.
Regulation of cell survival is a key part of the pathogenesis of multiple myeloma (MM). Jun N-terminal kinase (JNK) signaling has been implicated in MM pathogenesis, but its function is unclear. To elucidate the role of JNK in MM, we evaluated the specific functions of the two major JNK proteins, JNK1 and JNK2. We show here that JNK2 is constitutively activated in a panel of MM cell lines and primary tumors. Using loss-of-function studies, we demonstrate that JNK2 is required for the survival of myeloma cells and constitutively suppresses JNK1-mediated apoptosis by affecting expression of poly(ADP-ribose) polymerase (PARP)14, a key regulator of B-cell survival. Strikingly, we found that PARP14 is highly expressed in myeloma plasma cells and associated with disease progression and poor survival. Overexpression of PARP14 completely rescued myeloma cells from apoptosis induced by JNK2 knockdown, indicating that PARP14 is critically involved in JNK2-dependent survival. Mechanistically, PARP14 was found to promote the survival of myeloma cells by binding and inhibiting JNK1. Moreover, inhibition of PARP14 enhances the sensitization of MM cells to anti-myeloma agents. Our findings reveal a novel regulatory pathway in myeloma cells through which JNK2 signals cell survival via PARP14, and identify PARP14 as a potential therapeutic target in myeloma.
The JNKs are master protein kinases that regulate many physiological processes, including inflammatory responses, morphogenesis, cell proliferation, differentiation, survival and death. It is increasingly apparent that persistent activation of JNKs is involved in cancer development and progression. Therefore, JNKs represent attractive targets for therapeutic intervention with small molecule kinase inhibitors. However, evidence supportive of a tumour suppressor role for the JNK proteins has also been documented. Recent studies showed that the two major JNK proteins, JNK1 and JNK2, have distinct or even opposing functions in different types of cancer. As such, close consideration of which JNK proteins are beneficial targets and, more importantly, what effect small molecule inhibitors of JNKs have on physiological processes, are essential. A number of ATP-competitive and ATP-non-competitive JNK inhibitors have been developed, but have several limitations such as a lack of specificity and cellular toxicity. In this review, we summarize the accumulating evidence supporting a role for the JNK proteins in the pathogenesis of different solid and haematological malignancies, and discuss many challenges and scientific opportunities in the targeting of JNKs in cancer.
In the liver, the JNK cascade is induced downstream of TNF receptors (TNFRs) in response to inflammatory, microbial, and toxic challenges. Sustained activation of JNK triggers programmed cell death (PCD), and hepatocyte survival during these challenges requires induction of the NF-kappaB pathway, which antagonizes this activation by upregulating target genes. Thus, modulation of JNK activity is crucial to the liver response to TNFR-mediated challenge. The basis for this modulation, however, is unknown. Here, we investigated the role of the NF-kappaB target Gadd45b in the regulation of hepatocyte fate during liver regeneration after partial hepatectomy. We generated Gadd45b(-/-) mice and found that they exhibited decreased hepatocyte proliferation and increased PCD during liver regeneration. Notably, JNK activity was markedly increased and sustained in livers of Gadd45b(-/-) mice compared with control animals after partial hepatectomy. Furthermore, imposition of a Jnk2-null mutation, attenuating JNK activity, completely rescued the regenerative response in Gadd45b(-/-) mice. Interestingly, Gadd45beta ablation did not affect hepatotoxic JNK signaling after a TNFR-mediated immune challenge, suggesting specificity in the inducible hepatic program for JNK restraint activated during distinct TNFR-mediated challenges. These data provide a basis for JNK suppression during liver regeneration and identify Gadd45beta as a potential therapeutic target in liver diseases
NF-kappaB/Rel factors control programmed cell death (PCD), and this control is crucial to oncogenesis, cancer chemoresistance, and antagonism of tumor necrosis factor (TNF) alpha-induced killing. With TNFalpha, NF-kappaB-mediated protection involves suppression of the c-Jun-N-terminal kinase (JNK) cascade, and we have identified Gadd45beta, a member of the Gadd45 family, as a pivotal effector of this activity of NF-kappaB. Inhibition of TNFalpha-induced JNK signaling by Gadd45beta depends on direct targeting of the JNK kinase, MKK7/JNKK2. The mechanism by which Gadd45beta blunts MKK7, however, is unknown. Here we show that Gadd45beta is a structured protein with a predicted four-stranded beta-sheet core, five alpha-helices, and two acidic loops. Association of Gadd45beta with MKK7 involves a network of interactions mediated by its putative helices alpha3 and alpha4 and loops 1 and 2. Whereas alpha3 appears to primarily mediate docking to MKK7, loop 1 and alpha4-loop 2 seemingly afford kinase inactivation by engaging the ATP-binding site and causing conformational changes that impede catalytic function. These data provide a basis for Gadd45beta-mediated blockade of MKK7, and ultimately, TNFalpha-induced PCD. They also have important implications for treatment of widespread diseases.
NF-kappa B/Rel transcription factors control apoptosis, also known as programmed cell death. This control is crucial for oncogenesis, cancer chemo-resistance and for antagonizing tumour necrosis factor alpha (TNFalpha)-induced killing. With regard to TNFalpha, the anti-apoptotic activity of NF-kappa B involves suppression of the c-Jun N-terminal kinase (JNK) cascade. Using an unbiased screen, we have previously identified Gadd45beta/Myd118, a member of the Gadd45 family of inducible factors, as a pivotal mediator of this suppressive activity of NF-kappa B. However, the mechanisms by which Gadd45 beta inhibits JNK signalling are not understood. Here, we identify MKK7/JNKK2--a specific and essential activator of JNK--as a target of Gadd45 beta, and in fact, of NF-kappa B itself. Gadd45 beta binds to MKK7 directly and blocks its catalytic activity, thereby providing a molecular link between the NF-kappa B and JNK pathways. Importantly, Gadd45 beta is required to antagonize TNFalpha-induced cytotoxicity, and peptides disrupting the Gadd45 beta/MKK7 interaction hinder the ability of Gadd45 beta, as well as of NF-kappa B, to suppress this cytotoxicity. These findings establish a basis for the NF-kappa B control of JNK activation and identify MKK7 as a potential target for anti-inflammatory and anti-cancer therapy.
During inflammation, NF-kappaB transcription factors antagonize apoptosis induced by tumor necrosis factor (TNF)alpha. This antiapoptotic activity of NF-kappaB involves suppressing the accumulation of reactive oxygen species (ROS) and controlling the activation of the c-Jun N-terminal kinase (JNK) cascade. However, the mechanism(s) by which NF-kappaB inhibits ROS accumulation is unclear. We identify ferritin heavy chain (FHC)--the primary iron storage factor--as an essential mediator of the antioxidant and protective activities of NF-kappaB. FHC is induced downstream of NF-kappaB and is required to prevent sustained JNK activation and, thereby, apoptosis triggered by TNFalpha. FHC-mediated inhibition of JNK signaling depends on suppressing ROS accumulation and is achieved through iron sequestration. These findings establish a basis for the NF-kappaB-mediated control of ROS induction and identify a mechanism by which NF-kappaB suppresses proapoptotic JNK signaling. Our results suggest modulation of FHC or, more broadly, of iron metabolism as a potential approach for anti-inflammatory therapy.
INVITED EDITORIAL COMMENT (EXPERTS OPINIONS)
This is an invited editorial comment to express the opinions on a published article by Langiewicz and colleagues in the Journal of Hepatology.
A marked increase in the rate of glycolysis is a key event in the pathogenesis of hepatocellular carcinoma (HCC), the main type of primary liver cancer. Liver cirrhosis is considered to be a key player in HCC pathogenesis as it precedes HCC in up to 90% of patients. Intriguingly, the biochemical events that underlie the progression of cirrhosis to HCC are not well understood. In this study, we examined the expression profile of metabolic gene transcripts in liver samples from patients with HCC and patients with cirrhosis. We found that gene expression of glycolytic enzymes is up-regulated in precancerous cirrhotic livers and significantly associated with an elevated risk for developing HCC. Surprisingly, expression levels of genes involved in mitochondrial oxidative metabolism are markedly increased in HCC compared to normal livers but remain unchanged in cirrhosis. Our findings suggest that key glycolytic enzymes such as hexokinase 2 (HK2), aldolase A (ALDOA), and pyruvate kinase M2 (PKM2) may represent potential markers and molecular targets for early detection and chemoprevention of HCC.
In addition to coordinating immune and inflammatory responses, NF-kappaB/Rel transcription factors control cell survival. Normally, NF-kappaB dimers are sequestered in the cytoplasm by binding to inhibitory IkappaB proteins, and can be activated rapidly by signals that induce the sequential phosphorylation and proteolysis of IkappaBs. Activation of NF-kappaB antagonizes apoptosis or programmed cell death by numerous triggers, including the ligand engagement of 'death receptors' such as tumour-necrosis factor (TNF) receptor. The anti-apoptotic activity of NF-kappaB is also crucial to oncogenesis and to chemo- and radio-resistance in cancer. Cytoprotection by NF-kappaB involves the activation of pro-survival genes; however, its basis remains poorly understood. Here we report that NF-kappaB complexes downregulate the c-Jun amino-terminal kinase (JNK) cascade, thus establishing a link between the NF-kappaB and the JNK pathways. This link involves the transcriptional upregulation of gadd45beta/myd118, which downregulates JNK signalling induced by the TNF receptor (TNF-R). This NF-kappaB-dependent inhibition of the JNK pathway is central to the control of cell death. Our findings define a protective mechanism that is mediated by NF-kappaB complexes and establish a role for the persistent activation of JNK in the apoptotic response to TNF-alpha.
Articles
-
Lepore A, Kaci FN, Bubici C, Papa S. An Integrated Methodology to Quantify the Glycolytic Stress in Plasma Cell Myeloma in Response to Cytotoxic Drugs. Methods Mol Biol. 2675: 285-296 (2023).
-
Kaci FN, Lepore A, Papa S, Bubici C. Screening Kinase-Dependent Phosphorylation of Key Metabolic Reprogramming Regulators. Methods Mol Biol. 2675, 205-218 (2023).
-
Lepore A, Choy PM, Lee NCW, Carella MA, Favicchio R, Briones-Orta MA, Glaser SS, Alpini G, D'Santos C, Tooze RM, Lorger M, Syn WK, Papakyriakou A, Giamas G, Bubici C, Papa S. Phosphorylation and Stabilization of PIN1 by JNK Promote Intrahepatic Cholangiocarcinoma Growth. HEPATOLOGY. 74:2561-2579 (2021)
-
Arif E, Wang C, Swiderska-Syn MK, Solanki AK, Rahman B, Manka PP, Coombes JD, Canbay A, Papa S, Nihalani D, Aspichueta P, Lipschutz JH, Syn WK. Targeting myosin 1c inhibits murine hepatic fibrogenesis. Am J Physiol Gastrointest Liver Physiol. 320:G1044-G1053 (2021).
-
Flati V, Corsetti G, Papa S. Editorial: The Dynamic Interplay Between Nutrition, Autophagy and Cell Metabolism. Front Cell Dev Biol. 2021;9:684049.
-
Bubici C, Lepore A, Papa S. ASKing no more: the emerging role of DUSP12 in the regulation of hepatic lipid metabolism. HEPATOLOGY, 70:1091-1094 (2019).
-
Bubici C, Papa S. The Warburg Effect Regulation Under Siege: the Intertwined Pathways in Health and Disease. Front Cell Dev Biol. 7:80 (2019).
-
Papa S, Choy PM, Bubici C. The ERK and JNK pathways in the regulation of metabolic reprogramming. Oncogene 38: 2223-2240 (2019)
-
Lee NCW, Carella MA, Papa S and Bubici C. High Expression of Glycolytic Genes in Cirrhosis Correlates With the Risk of Developing Liver Cancer. Front. Cell Dev. Biol. 6:138 (2018).
-
Papa S, Bubici C. Feeding the Hedgehog: A new meaning for JNK signalling in liver regeneration. J Hepatol. 69: 572-574 (2018).
-
Manka P, Coombes JD, Boosman R, Gauthier K, Papa S, Syn WK. Thyroid hormone in the regulation of hepatocellular carcinoma and its microenvironment. Cancer Lett. 419: 175-186 (2018).
-
Verzella D, Bennett J, Fischietti M, Thotakura AK, Recordati C, Pasqualini F, Capece D, Vecchiotti D, D'Andrea D, Di Francesco B, De Maglie M, Begalli F, Tornatore L, Papa S, Lawrence T, Forbes S, Sica A, Alesse E, Zazzeroni F, Franzoso G. GADD45β loss ablates innate immunosuppression in cancer. Cancer Res. 78: 1275–1292 (2018).
-
Papa S and Bubici C. Linking apoptosis to cancer metabolism: another missing piece of JuNK. Mol Cell Oncol, 3: e1103398 (2016).
-
Iansante V, Choy PM, Fung SW, Liu Y, Chai J-G, Dyson J, Del Rio A., D’Santos C, Williams R, Chokshi S, Anders RA, Bubici C and Papa S. PARP14 promotes the Warburg effect in hepatocellular carcinoma by inhibiting JNK1-dependent PKM2 phosphorylation and activation. Nat Commun, 6: 7882 (2015).
-
Coombes J, Swiderska-Syn M, Dollé L, Reid D, Eksteen B, Claridge L, Briones-Orta MA, Shetty S, Oo YH, Riva A, Chokshi S, Papa S, Mi Z, Kuo PC, Williams R, Canbay A, Adams DH, Diehl AM, van Grunsven LA, Choi SS, Syn WK. Osteopontin neutralization abrogates the liver progenitor cell response and fibrogenesis in mice. Gut, 64: 1120-1131 (2015).
-
Bubici C and Papa S. JNK signalling in cancer: in need of new, smarter therapeutic targets. Br J Pharmacol, 171: 24-37 (2014).
-
Barbarulo A, Iansante V, Chaidos A, Naresh K, Rahemtulla A, Franzoso G, Karadimitris A, Haskard DO, (*)Papa S, Bubici C. Poly(ADP-ribose) polymerase family member 14 (PARP14) is a novel effector of the JNK2-dependent pro-survival signal in multiple myeloma. Oncogene, 32: 4231-4242 (2013).
-
Papa S., Bubici C., Zazzeroni F. & Franzoso G. Mechanisms of Liver Disease: The crosstalk between the NF-κB and JNK pathways. Biol Chem, 390: 965-976 (2009).
-
Svensson CI., Inoue T., Hammaker D., Fukushima A., Papa S., Franzoso G., Schett G., Corr M., Boyle D. & Firestein GS. Deficient Gadd45β in rheumatoid arthritis: Enhanced synovitis through JNK signalling. Arthritis Rheum, 60: 3229-3240 (2009).
-
Tornatore L., Montil SM., Marasco D., Dathan N., Vitale RM., Benedetti E., Pedone C., Papa S., Franzoso G. & Ruvo M. Gadd45β dimerization does not affect MKK7 binding. Adv Exp Med Biol, 611: 367-368 (2009).
-
Mauro C., Zazzeroni F., Papa S., Bubici C. & Franzoso G. The NF-κB transcription factor pathway as a therapeutic target in cancer: Methods for detection of NF-κB activity. Methods Mol Med, 512: 169-207 (2009).
-
Tumanov AV, Koroleva EP, Christiansen PA, Khan MA, Ruddy MJ., Burnette B., Papa S, Franzoso G., Nedospasov S., Fu YX. & Anders RA. T cell-derived lymphotoxin regulates liver regeneration. Gastroenterology, 136: 694-704 (2009).
-
Liu B., Suyeoka G., Papa S., Franzoso G. & Neufeld AH. Growth arrest and DNA damage protein 45β (Gadd45β) protects retinal ganglion cells from injuries. Neurobiol Dis, 33: 104-110 (2009).
-
Papa S., Zazzeroni F., Fu YX., Bubici C., Alvarez K., Dean K., Christiansen P., Anders RA. & Franzoso G. Gadd45β promotes hepatocyte survival during liver regeneration in mice by modulating JNK signaling. J Clin Invest, 118: 1911-1923 (2008).
-
Tornatore L., Marasco D., Dathan N., Vitale RM., Pedone C, Papa S., Franzoso G., Ruvo M & Monti SM. Gadd45β forms a homodimeric complex that binds tightly to MKK7. J Mol Biol, 378: 97-111 (2008).
-
Papa S., Monti SM., Vitale RM., Bubici C., Jayawardena S., Alvarez K., De Smaele E., Dathan N., Pedone C., Ruvo M. & Franzoso G. Insights into the structural basis of the Gadd45β-mediated inactivation of the JNK Kinase, MKK7/JNKK2. J Biol Chem, 282: 19029-19041 (2007).
-
Kuntzen C., Zazzeroni F., Pham CG., Papa S., Bubici C., Knabb JR., & Franzoso G. A method for isolating prosurvival targets of NF-κB/Rel transcription factors. Methods Mol Biol, 399: 99-124 (2007).
-
Pham CG., Bubici C., Zazzeroni F., Knabb JR., Papa S. , Kuntzen C & Franzoso G. Upregulation of Twist-1 by NF-κB blocks cell killing induced by chemotherapeutic drugs. Mol Cell Biol, 27: 3920-3935 (2007).
-
Centeno C., Repici M., Chatton J-Y., Riederer B.M., Bonny C., Nicod P., Price M., Clarke PGH., Papa S., Franzoso G. & Borsello T. Protective features of the cell permeable JNK Inhibitor peptide against excitotoxicity: identification of a new key modulator of a new key modulator of a death signaling pathway in cortical neurons. Cell Death Diff, 14: 240-253 (2007).
-
Bubici C., Papa S., Dean K. & Franzoso G. Mutual cross-talk between reactive oxygen species (ROS) and NF-κB: molecular basis and biological significance. Oncogene, 25: 6731-6748 (2006).
-
Larsen CM., Dossing MG., Papa S., Franzoso G., Billestrup N. & Mandurp-Poulsen T. Growth arrest- and DNA-damage-inducibile 45β gene inhibits c-Jun N-terminal kinase and extracellular signal-regulated kinase and decrease IL-1β-induced apoptosis in insulin-producing INS-1E cells. Diabetologia, 49: 980-989 (2006).
-
Papa S., Bubici C., Zazzeroni F., Pham CG., Kuntzen C., Knabb JR., Dean K. & Franzoso G. The NF-κB-mediated control of JNK cascade in the antagonism of programmed cell death in health and disease. Cell Death Diff, 13: 712-729 (2006).
-
Mittal A., Papa S., Franzoso G. & Sen R. NF-kB-dependent regulation of the timing of activation-induced cell death of T lymphocytes. J Immunol, 176: 2183-2189 (2006).
-
Bubici C., Papa S., Pham CG., Zazzeroni F. & Franzoso G. The NF-kB-mediated control of ROS and JNK signaling. Histol Histopathol, 21: 69-80 (2006).
-
Pham CG., Papa S., Bubici C., Zazzeroni F. & Franzoso G. In the crosshairs: NF-κB targets the JNK signaling cascade. Curr Med Chem, 4: 569-576 (2005).
-
Papa S., Bubici C., Pham CG., Zazzeroni F. & Franzoso G. NF-κB meets ROS: an “iron-ic” encounter. Cell Death Diff, 12: 1259-1262 (2005).
-
Pham C.G., Papa S., Bubici C., Zazzeroni F. & Franzoso G. Oxygen JNKies: Phosphatates overdose on ROS. Dev Cell, 8: 452-454 (2005).
-
Bubici C., Papa S., Pham CG., Zazzeroni F. & Franzoso G. NF-κB and JNK: an intricate affair. Cell Cycle, 3: 1524-1529 (2004).
-
Pham CG., Bubici C., Zazzeroni F#, Papa S#, Jones J., Alvarez K., Jayawardena S., De Smaele E., Cong R., Beaumont C., Torti F.M., Torti S.V. & Franzoso G. Ferritin heavy chain upregulation by NF-κB inhibits TNFα-induced apoptosis by suppressing reactive oxygen species. Cell 119: 529-542 (2004).
-
Papa S., Zazzeroni F., Pham CG., Bubici C. & Franzoso G. Linking JNK signaling to NF-κB: a key to survival. J Cell Sci, 117: 5197-5208. (2004).
-
Papa S., Zazzeroni F., Bubici C., Jayawardena S., Alvarez K., Matsuda S., Nguyen DU., Pham CG., Nelsbach AH., Melis T., De Smaele E., Tang WJ., D’Adamio L. & Franzoso G. Gadd45β mediates the NF-κB suppression of JNK signaling by targeting MKK7/JNKK2. Nat Cell Biol, 6: 146-153 (2004).
-
Zazzeroni F., Papa S., Algeciras-Schimnich A., Alvarez K., Melis T., Bubici C., Majewski N., Hay N., De Smaele E., Peter M.E. & Franzoso G. Gadd45β mediates the protective effects of CD40 costimulation against Fas-induced apoptosis. Blood, 102: 3270-3279 (2003).
-
Zazzeroni F., Papa S., De Smaele E. & Franzoso G. Cell survival and a Gadd45-factor deficiency. Nature, 424: 742 (2003).
-
Franzoso G., Zazzeroni F. & Papa S. JNK: A killer on a transcriptional leash. Cell Death Diff, 10: 13-15 (2003).
-
Jin R., De Smaele E., Zazzeroni F., Nguyen D.U., Papa S., Jones J., Cox C., Gelinas C. & Franzoso G. Regulation of the gadd45β promoter by NF-κB. DNA Cell Biol, 21: 491-503 (2002).
-
De Smaele E., Zazzeroni F., Papa S., Nguyen DU., Jin R., Jones J., Cong R. & Franzoso G. Induction of gadd45β by NF-κB downregulates pro-apoptotic JNK signaling. Nature, 414: 308-313 (2001).
Conference proceedings
-
Papa S(*), Lee NCW, Bubici C. Deciphering preventive and prognostic biomarkers of liver cancer
NCRI Cancer Conference (2018) https://bit.ly/2CIpyMl -
Briones MA, Coombes JD, Mellone M, Rispoli R, Tolosa E, Manka PP, Kitamura N, Quaglia A, Canbay A, Alpini G, Glaser SS, Williams R, Papa S, Fernandez-Zapico ME, Syn W-K. The role of osteopontin isoforms in cholangiocarcinoma. Hepatology 64 (Suppl 1): 235A-235A (2016). doi:10.1002/hep.28796.
-
Iansante V, Choy PM, Fung SW, Liu Y, Chai J-G, Dyson J, Del Rio A., D’Santos C, Williams R, Chokshi S, Anders RA, Bubici C and (*)Papa S Linking apoptosis to altered metabolism in cancer cells. NCRI Cancer Conferences: B229 (2015) http://bit.ly/2tzital
-
Choy PM, Sufi J, Glaser S, Alpini G, Heaton N, Williams R, Quaglia A, Syn W-K, Bubici C, (*)Papa S. Inhibition of MAPK signalling promotes cell cycle arrest and sensitises intrahepatic cholangiocarcinoma cells to chemotherapy. Gut 64(Suppl 1): A458.2-A459 (2015).
-
Iansante V, Choy PM, Chokshi S, Williams R, Anders RA, Bubici C, (*)Papa S. Increased aerobic glycolysis is associated with poor outcome and suppression of apoptosis in human liver cirrhosis and HCC. J Hepatol 62, S427 (2015).
-
Coombes JD, Swiderska-Syn M, Dollé L, Reid DT, Eksteen B, Claridge LC, Briones-Orta MA, Shishir S, Oo YH, Riva A, Chokshi S, Papa S, Mi Z, Kuo PC, Williams R, Canbay A, Adams DH, van Grunsven LA, Diehl AM, Choi SS, Syn W-K. Osteopontin neutralization abrogates the liver progenitor cell response and fribrogenic outcomes in mice. J Hepatol 60, S215-S359 (2014).
-
Briones-Orta MA, Coombes JD, Kitamura N, Manka PP, Williams R, Canbay A, Papa S, Wing-Kin Syn W-K. Osteopontin isoforms are upregulated in human cholangiocarcinoma cells and modulate levels of the TGF-beta repressor, SnoN. Hepatology 60, 818A-818A (2014).
-
Iansante V, Choy PM, Fung SW, Chai J-G, Dyson J, Liu Y, Williams R, Chokshi S, Anders RA, Bubici C, (*)Papa S. Upregulation of a novel protein in HCC enhances cancer cell survival by suppressing specific apoptotic effectors. J Hepatol 1 (60), S89 (2014).
-
Coombes JD, Claridge LC, Swiderska-Syn M, Briones-Orta MA, Younis R, Shah H, Papa S, Diehl AM, Eksteen B, Canbay A, Syn W-K. Osteopontin enhances liver progenitor cell responses in progressive Nonalcoholic Steatohepatitis. Hepatology 58, 535A (2013).
-
Iansante V, Zen Y, Barbarulo A, Starling C, Chokshi S, Heaton N, Williams R, Bubici C, Quaglia A, (*)Papa S. MAPK signalling regulates the development of a cholangiocellular phenotype from HCC in post-TACE liver transplants. Gut 61(Suppl 2), A416 (2012).
-
Iansante V, Zen Y, Barbarulo A, Starling C, Chokshi S, Heaton N, Williams R, Bubici C, Quaglia A, (*)Papa S. Mixed-phenotype hepatocellular carcinoma in liver transplants after use of transaerterial chemoembolization (TACE) is associated with activation of Mitogen-Activated Protein Kinase (MAPK) signaling pathway. J Hepatol 56, S117 (2012).
-
De Smaele E, Zazzeroni F, Papa S, Nguyen DU, Jin RG, Jones J, Cong R, Franzoso G. Induction of gadd45 beta by NF-kappa B down-regulates pro-apoptotic JNK signaling. FASEB J 16 (4), A139 (2002).
-
Papa S., Zazzeroni F., Bubici C., Monti S., Fu Y-X, Anders RA., Ruvo M. and Franzoso G. Structural basis for the Gadd45β-mediated inactivation of MKK7. EMBO Workshop – October 18-21, 2008. Capri, Italy
-
Tornatore L., Monti S. M., Marasco D., Dathan N., Vitale R. M., Benedetti E., Pedone C., Papa S., Franzoso G., Ruvo M. Gadd45β Dimerization does not affect MKK7 binding. 20th American-Peptide-Society Symposium – June 26-30, 2007. Montreal, Canada.
-
Pham CG., Zazzeroni F., Knabb JR., Bubici C., Kuntzen C., Papa S. & Franzoso G. Upregulation of Twist-1 by NF-κB blocks cell killing induced by chemotherapeutic drugs. Keystone Symposia: NF-κB: 20 Years on the road from Biochemistry to Pathology – March 23-28, 2006. Banff, Alberta, Canada.
-
Papa S., Bubici C., Monti S.M., Dathan N., Jayawardena S., Vitale R.M., Saviano M., Alvarez K., De Smaele E., Pedone C., Ruvo M. & Franzoso G. Gadd45β blocks activity of the JNK kinase MKK7/JNKK2 and apoptosis through a discrete domain. Keystone Symposia: NF-κB: 20 Years on the road from Biochemistry to Pathology – March 23-28, 2006. Banff, Alberta, Canada.
-
Zazzeroni F., Papa S., Algeciras-Schimnich A., Alvarez K., Melis T., Bubici C., Majewski N., Hay N., De Smaele E., Peter M., Franzoso G. Gadd45β mediates the protective effects of CD40 co-stimulation against Fas-induced apoptosis. Keystone Symposia: NF-κB: Biology and Pathology – January 11-16, 2004. Snowbird, Utah, USA.
-
Papa S., Zazzeroni F., Bubici C., Jayawardena S., Alvarez K., Matsuda S., Nguyen DU., Pham CG., Nelsbach AH., Melis T., De Smaele E., Tang W-J., D'Adamio L., Franzoso G. Gadd45β mediates the NF-κB suppression of JNK signaling by targeting MKK7/JNKK2. Keystone Symposia: NF-κB: Biology and Pathology – January 11-16, 2004. Snowbird, Utah, USA.
-
De Smaele E., Zazzeroni F., Papa S., Nguyen DU., Jin R., Jones J., Cong R., Cox C., Gelinas C., Franzoso G. A novel mechanism of NF-κB-mediated cytoprotection: the activation of gadd45β to terminate pro-apoptotic JNK signaling. 4th FISV Conference (Federazione Italiana Scienze della Vita) September 20-23, 2002. Riva del Garda (TN) Italy.
-
De Smaele E., Zazzeroni F., Papa S., Nguyen DU., Jin R., Jones J., Cong R., Franzoso G. Induction of gadd45β by NF-κB downregulates pro-apoptotic JNK signalling. Keystone Symposia: NF-κB: Bench to Bedside – February 25 - March 3, 2002. Keystone, Colorado, USA.